Research Funding & Impact
ABTA-Funded Research Projects
2023 Research Funding
Guided by our mission, and advised by a multidisciplinary team of experts, the ABTA is dedicated to funding research that has the potential to change the lives of people affected by brain tumors. We also aim to “seed the field” with promising up-and-coming researchers in the brain tumor space.
- The ABTA awarded $1,474,000 in grants to 24 new projects
- $600,000 funded 3 Research Collaboration Grants
- $450,000 funded 9 Discovery Grants
- $400,000 funded 4 Basic Research Fellowships
- $24,000 funded 8 Medical Student Summer Fellowships
- The 2023 funded projects span multiple tumor types:
- Glioblastomas
- Low Grade Gliomas
- Meningiomas
- Brain Metastasis
- Research areas of focus:
- Immunology/Immunotherapy
- Viral & Gene Therapies
- Epigenetics
- Imaging
- Biomarkers
- Aging
The ABTA has contributed over $35.5 million to brain tumor research since we began funding research in 1976.
Jack & Fay Netchin Medical Student Summer Fellowship
A Medical Student Summer Fellowship is a three month, mentor-guided summer research experience, intended to motivate talented medical students to pursue a career in neuro-oncology research.

Sangami Pugazenthi, BA
Institution: Washington University in St. Louis
Mentor: Albert Kim, MD, PhD
Tribute: In honor of Paul Fabbri
Grade 2 meningiomas (G2M) are often treated with surgical resection followed by radiation, especially in the case of recurrence. However, the mechanisms underlying radiation resistance in G2Ms remain to be identified. Moreover, we lack biomarkers to distinguish G2Ms that will respond well to radiotherapy from those that do not. In this study we perform histological and molecular analysis of a large cohort tumors from G2M patients, to identify predictors of radiation resistance. Using these data and a model of radiation therapy, we learned that radiation resistance in G2Ms is likely driven by the combination of mutations in the tumor suppressor gene, NF2, and the hypoxia (low oxygen) that occurs with tumor necrosis. Analysis of patient tumor data revealed downregulation of cell death signals and upregulation of proliferation in NF2-deficient and hypoxic cells, which were validated with functional assays. These pathways may identify tumors resistant to radiation and represent therapeutic targets that in the future could improve outcomes in patients with radiation resistant G2Ms.
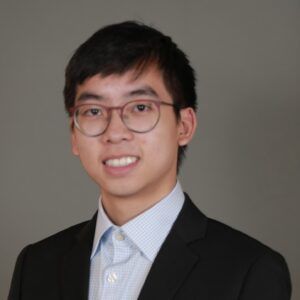
Michael Chang, BS
Institution: Johns Hopkins University School of Medicine
Mentor: Karisa Schreck, MD, PhD
Tribute: In memory of Brian Bouts
Glioblastoma is an aggressive brain cancer for which there is no cure. From diagnosis, patients typically have anywhere from months to a couple years to live, and very few treatment options exist. A promising new class of drugs, called targeted therapy, seeks to change this. Targeted therapies work by targeting DNA and protein changes unique to a patient’s cancer. One of these potential targets is the neurofibromin (NF1) gene. This gene codes for a protein that prevents cancer cell growth. Many glioblastomas either possess low levels of the NF1 protein or a defective version of NF1. This is a target that could potentially benefit many future patients. Unfortunately, no method exists to reliably identify defective NF1, making it difficult to conduct the clinical trials needed to bring an NF1-targeted therapy to the bedside. The goal of this project is to establish a method for identifying loss of NF1 in glioblastoma.
We assembled a panel of 73 human glioblastoma tissue samples. We searched the panel for several proteins and evaluated the pattern of gene mutations and protein expression levels within each of the tumors. We found that one of our selected antibodies against NF1 was satisfactory in assessing levels of the NF1 protein. Tumors with low levels of NF1 protein tended to have common patterns of DNA mutations, and patients with these same tumors also tended to have shorter overall survival. These findings are valuable first steps to developing a reliable and clinically applicable method for identifying which glioblastoma patients have NF1 loss and may be good candidates for targeted therapy.
Himanshu Dashora, BS
Institution: Cleveland Clinic
Mentor: Jennifer Yu, MD, PhD
Tribute: Fully supported by Southeastern Brain Tumor Foundation
Glioblastoma (GBM) is a CNS tumor derived from glial cells. It is the most common and fatal primary malignant brain tumor in adults with a median survival of 15 months. The cells that serve as the originator of GBM are known as glioma stem cells (GSC). Furthering our understanding of GSCs is a crucial step in developing targeted treatments for GBM. Previous work, from our laboratory and others, has shown that GSCs reside in distinct locations within the tumor, including low-oxygen regions. In this project, I utilized sequencing methods known as RNA-seq and RIBO-seq to understand how GSCs may adapt to low-oxygen in their local environment. By combining these two sequencing methods, I was able to see how the tumor cells changed the genes that are used in response to low oxygen, both as the DNA was transcribed into mRNA and the mRNA translated into protein. I found that, in response to the low oxygen environment, the cells altered the translation of mRNAs to proteins by more than 60%. I also identified the Integrated Stress Response (ISR), and the coordinated of behavior of ISR-members GCN2/eIF2a/ATF4, as a potential signaling pathway for further study. In our patient-derived GSCs, I demonstrated that a gene knock-down of GCN2 results in reduced cancer stem cell viability, suggesting that it may be a promising target. Further research will show whether this pathway can be targeted as a potential new treatment for GBM patients.

Jakub Jarmula, BA
Institution: Cleveland Clinic
Mentor: Justin Lathia, PhD
Tribute: In honor of Bats for Brains
Meningioma is the most common tumor within the skull, arising from the tissue surrounding the brain. While many meningiomas are non-cancerous, up to 20% are pre-cancerous or cancerous (i.e., high-grade). Patients with high-grade meningioma have very bad outcomes despite treatment with surgery and radiation. There are currently no medicines for high-grade meningioma, such as chemotherapy or immunotherapy. Immunotherapy, for example anti-PD1 immunotherapy, helps the immune system target tumors. Research has found that tumors change their local surroundings to weaken the immune system, leading to tumor growth and spread. Interleukin-1-beta (IL1b) is a protein produced by tumors that weakens the immune system. Blocking IL1b can restore the function of the immune system against tumors. Research in other cancers has found that IL1b inhibition works well for females, but not males. The aim of this research project was to investigate whether females and males with high-grade meningioma have different responses to IL1b inhibition combined with anti-PD1 immunotherapy. This study was designed to be performed with patient-derived samples of high-grade meningioma. Although there were difficulties with tissue samples for these planned experiments, future work will be focused on optimizing conditions for these samples to study this combination therapy. We continue to pursue this work in the hopes that the findings from this research project will inform the development of new medicines for patients with high-grade meningioma.
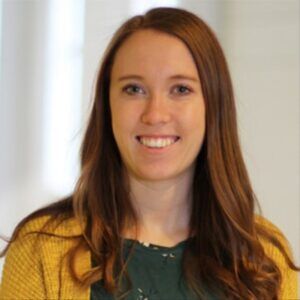
Jenna Koenig, BS
Mentor: Karen Pollok, PhD
Institution: Indiana University
Tribute: Fully supported by BrainUp
Treatment options remain limited for glioblastoma (GBM) as temozolomide (TMZ) chemotherapy is the mainstay medication given to patients despite risks for many side effects and development of resistance to the drug and thus tumor recurrence. TMZ works by damaging DNA in cancer cells, leading to cell death. In healthy cells, DNA damage can be repaired by the cell to survive small injuries. GBM cells, however, can send these DNA repair systems into overdrive to survive treatment with TMZ and continue to grow as tumors. To improve the effectiveness of TMZ in killing GBM cells and reducing tumor cell growth, we studied the use of two drugs that specifically target systems these cells use to repair DNA. Targeting the abnormal DNA repair machinery used specifically in cancer cells means drugs are less likely to harm healthy cells, minimizing treatment side effects. GBM cells use multiple DNA repair systems to survive TMZ, such that attacking DNA repair from multiple angles helps ensure cell death following TMZ. We found that a three-drug combination of TMZ with drugs blocking two key regulators of survival and DNA repair, MDM2 and AKT, improves the effectiveness of TMZ by stopping cell growth and triggering cell death in TMZ-resistant GBM cells. This triple combination is a promising option for improving TMZ effectiveness in GBM and further study of how GBM cells respond to this therapy using animal model systems will be the next step in developing this treatment option for patients.

Jayson Nelson, BS
Institution: The University of Utah
Mentor: Randy Jensen, MD, PhD
Tribute: In memory of Jeffrey Michael Tomberlin
Glioblastoma is the most common form of brain cancer in adults with a median survival time of just 12-15 months. The current standard of care for glioblastoma is surgical resection followed by radiation and chemotherapeutic agents. One challenge in treating glioblastoma is the difficulty of facilitating a gradual release of therapeutic agents localized to the cancerous site. Our lab aims to use injectable hydrogels that will slowly release the therapeutics at the site of the tumor. We hypothesize that this will in turn provide a more effective and long-lasting treatment against the tumor, resulting in longer median survival times. The first phase in achieving this objective is to determine the appropriate concentration of therapeutic within the hydrogel environment to ensure an effective treatment for glioblastoma. The results of this project will allow us to identify a concentration of therapeutic within the hydrogel that inhibits further growth of glioblastomas.

Minh Nguyen, BS
Institution: University of California, San Francisco
Mentor: David Raleigh, MD, PhD
Tribute: In memory of Rose Digangi
Meningiomas are among the most common brain tumors. The current standard of care for meningioma treatment involves surgery and radiation. However, many more aggressive meningiomas may not respond to therapy, and there is a need to improve our ability to identify and understand these tumors. This project aimed to better classify chromosomal alterations in meningioma and mechanisms that might underly more aggressive behavior. Copy number variants (CNVs), large gains or losses of chromosomes, are known to affect the behavior of meningioma and other cancers. We found that CNVs best predicted meningioma recurrence or patient death at different sizes depending on the chromosome, likely because they affect different genes and pathways. We also found certain CNVs that are implicated in meningioma, such as loss of chromosome segments 22q or 1p, have worse prognosis when they occur together, and that one may precede the other. These are novel findings that may impact the use of CNVs as a marker of risk stratification for meningiomas that have been biopsied or surgically removed. We also began laboratory experiments in which meningioma cells were exposed to either chemotherapy or radiotherapy, and we will be sequencing the resulting DNA to assess for mechanisms of resistance that can inform treatment planning.
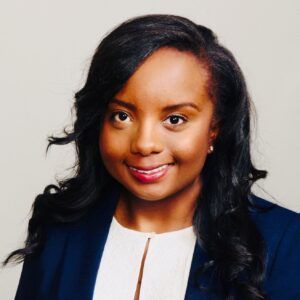
Gabrielle Price, MSc
Institution:
Mentor: Dolores Hambardzumyan, PhD
Tribute: Fully supported by The Gladiator Project
Diffuse midline glioma (DMG) is the most devastating pediatric malignant tumor arising in the brainstem, a region of the brain controlling breathing, blood pressure, and heart rate. Currently, there is no cure for DMG, and treatments have failed because of the unremitting nature of the disease. Photodynamic therapy (PDT), a therapy that activates molecules to cause cell death and reorganize the tumor microenvironment, is known to have profound inflammatory effects on tumor cells and synergize with MEK inhibition (MEKi). Our goal is to understand how PDT activated by 5-aminolevulinic acid (5-ALA-PDT), an intraoperative imaging agent for brain tumor surgery, causes the tumor cells to alter the use of genetic information and how it affects the tumor microenvironment. ]We determined that the combination is effective in improving DMG survival in mice with DMG tumors. Since signals from neighboring tumor cells or the non-tumor microenvironment can influence a tumor cell’s invasive behavior, we found that the treatment combination alters the microenvironment and creates an antitumor response. These findings demonstrate that 5-ALA-PDT together with MEK inhibitors produce moderate antitumor effects by inducing tumor cell death, promoting neural stem cell differentiation, and modifying the tumor microenvironment.
2023 Newly Awarded Grants
Research Collaboration Grants
The Research Collaboration Grant is a two-year, $200,000 grant, to support interdisciplinary team science projects that combine resources to streamline accelerate progress in the brain tumor field.
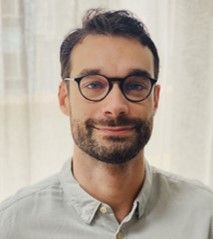

Federico Gaiti, PhD
Co-PI: Gelareh Zadeh, MD, PhD, FRCSC
Institution: University Health Network
Co-PI Institution: University of Toronto
Tribute: In memory of George Surgent
Tumors continuously evolve into more aggressive and treatment-resistant forms. Brain tumors, such as gliomas, illustrate the dilemma of cancer evolution. Despite standard-of-care treatments, these tumors inevitably recur, with an average survival rate of about one year, thereby underscoring an urgent need for novel treatments.
Our preliminary findings suggest that these incurable brain tumors exhibit extensive diversity within and harbor multiple types of malignant cells, presenting a significant unmet therapeutic challenge. Therefore, identifying the key molecular factors that drive this cellular diversity and promote malignant transformation is a crucial goal for neuro-oncology and represents a prime opportunity for intervention. However, we currently lack a comprehensive understanding of the primary molecular factors that contribute to glioma diversity and malignant transformation.
In this project, we aim to develop and apply innovative genomic and experimental technologies to glioma samples collected at diagnosis and upon recurrence following chemotherapy and radiotherapy. This data will enhance our understanding of aggressive malignant transformation, tumor progression, and therapeutic resistance. These studies are expected to lead to novel treatment strategies for this devastating cancer, improving patient outcomes. Additionally, this work will generate new tools for investigating tumor evolution across other types of brain tumors.


Oliver Jonas, PhD
Co-PI: Shawn Hervey-Jumper, MD
Institution: Brigham and Women’s Hospital
Co-PI Institution: University of California, San Francisco
Tribute: Partially supported by StacheStrong
This project utilizes an implantable microdevice to measure multiple drug responses directly within the tumor of glioblastoma patients. We have developed a miniaturized implant, roughly the size of a grain of rice, that is implanted into the patient, remains inside the tumor for 3 days, and is then removed during a standard surgical tumor resection. The implant releases microdoses of 20 different drugs into different regions of the tumor. After surgery, we examine the tumor response to each of the treatments independently using a range of cutting-edge spatial biology techniques. This study represents the first time that measurements of multiple drug responses from inside the tumor of the same patient are realized. We expect that the resulting data set will allow us to systematically understand drug response and resistance pathways to established and novel treatment options. The goal is to rationally select highly effective combination treatment regimens that are based on each patient’s actual tumor biological response to the range of available drugs.

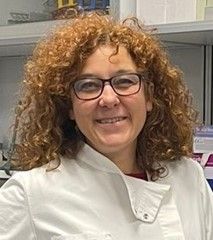
Derek Wainwright, PhD
Co-PI: Pilar Sanchez-Gomez, PhD
Institution: Loyola University of Chicago
Co-PI Institution: Instituto de Salud Carlos III
Tribute: Partially supported by BrainUp
Older adults ≥65 years of age represent more than 70% of all new glioblastoma (GBM) diagnoses. Older adults with GBM tend to experience significantly worse overall survival as compared to their younger GBM patient counterparts. However, the biological underpinnings for the dismal outcomes in older GBM patients is poorly understood. Our study aims to determine how the older adult brain macroenvironment that’s, outside of the bulk GBM mass, contributes to worse outcomes in older adults with GBM . The remarkable improvements in healthcare has led to a world-wide population that is increasingly entering an age range associated with unprecedented increases in cancer incidence that will have important economic and public health consequences. Historically however, most older adults with GBM have been excluded from participating in clinical trials. Thus, more precision medicine approaches that are specifically tailored to older adults are necessary for understanding how best to improve the outcomes in this underserved GBM patient population. As direct result of our studies, we believe that our discoveries will improve treatment-related outcomes for older adults with GBM. By understanding age-dependent factors that decrease survival, we also believe that our work will indirectly improve outcomes for middle-aged and younger GBM patients that also eventually suffer from the hyper-aging effects caused by GBM.
Basic Research Fellowship
The Basic Research Fellowship is a two-year, $100,000 grant, awarded to post-doctoral fellows who are mentored by established and nationally-recognized experts in the neuro-oncology field.
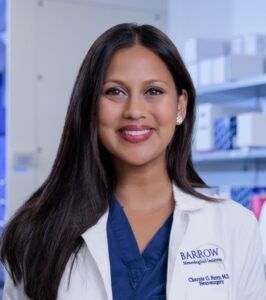
Charuta Furey, MD
Institution: Barrow Neurological Institute, St. Joseph Medical Center
Mentors: Nader Sanai, MD
Tribute: In honor of Joel A. Gingras, Jr.
Glioblastoma (GBM) is the most common type of malignant brain tumor in adults and remains incurable: most patients die within two years of diagnosis. Tracking glioblastoma evolution in response to experimental therapies is crucial to developing effective treatment breakthroughs. This study proposes a new method of liquid biopsy that involves collecting cerebrospinal fluid (CSF) from a reservoir in the tumor cavity during outpatient clinic visits. This minimally invasive technique allows researchers to isolate and examine circulating tumor DNA and GBM cells over time, which can help understand how the tumor evolves and develops resistance to therapy. If successful, this study will be the first to use serial CSF biopsies to prospectively monitor GBM evolution in response to a novel DNA Damage Response inhibitor. Findings from this study will help us better understand GBM resistance mechanisms and can be leveraged to create better combination therapies. Additionally, this minimally invasive liquid biopsy technique has potential clinical utility in helping us predict treatment response, differentiate pseudoprogression, and even catch recurrent disease before traditional MRI surveillance methods.

Juyeun Lee, PhD
Institution: Cleveland Clinic
Mentor: Justin Lathia, PhD
Tribute: Fully supported by Tap Cancer Out
Glioblastoma, the most aggressive primary brain tumor, affects more men than women, and men generally have worse outcomes. These differences between men and women are due in part to how the immune system responds to the brain tumor. Immunotherapy, which aims to enhance a patient’s own immune system to fight against the tumor, has worked well in some other types of cancer, however, they haven’t been successful in treating glioblastoma. Therefore, it is important to discover a new way to improve the current therapeutics for glioblastoma patients. Our laboratory found that male and female immune cells, specifically T cells which can kill cancer cells, responded differently to the cancer, with male T cells becoming dysfunctional more quickly than female cells.
To understand why male and female T cells are different, I want to examine the cells’ energy factories, called mitochondria, and how they work differently between men and women, as mitochondria function is crucial in regulating T cell function. I hypothesize that differences in mitochondria could lead to faster T cell dysfunction (also referred to as exhaustion) and contribute to faster cancer growth in men. To investigate this further, I will study how the mitochondria in T cells behave in male and female tumors. I will also test a new treatment that could help boost the mitochondria specifically in T cells. These studies could help develop new treatments that are tailored to men and women’s different immune responses to the cancer.

Jun Nishida, PhD
Institution: Dana-Farber Cancer Institute
Mentor: Kornelia Polyak, MD, PhD
Tribute: Fully supported by Breast Cancer Research Foundation
Brain metastasis is a challenging clinical problem leading to the shortest survival among metastatic breast cancer patients, yet therapeutic options are very limited. Brain metastases of different cancers preferentially grow in specific parts of the brain. For example, brain metastases of melanoma frequently occur in the frontal regions while those of breast cancer can form everywhere but are most likely found in the back of the brain. The significance of these differences has not been studied and all patients are treated the same way regardless of the location in the brain.
The levels of nutrients in the brain are very different from those of other organs and even within the brain each region can be different. Thus, cancer cells must adapt to this special environment to be able to form tumors. In my preliminary studies I identified candidate molecules in breast cancer cells that regulate adaptation to the brain, including proteins that can be therapeutic targets. In this proposal I will further investigate how differences in nutrient levels in different parts of the brain are preferred by cancer cells with different properties and how we can exploit this to improve the treatment of patients with brain metastases. The mechanisms I identified also play a role in the growth of pediatric gliomas. Thus, the successful completion of my proposed study will facilitate the understanding and treatment of not only brain metastatic cancer patients, but also children with highly aggressive brain tumors.
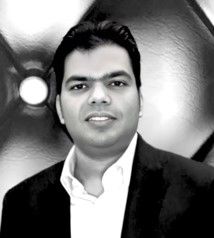
Rakesh Trivedi, PhD
Institution: University of Texas M.D. Anderson Cancer Center
Mentor: Krishna Bhat, PhD
Tribute: Fully supported by Tap Cancer Out
The most aggressive form of primary malignant brain tumor is glioblastoma, with a 5-year survival rate of less than 5%. Monitoring glioblastoma progression and treatment response with the current brain scanning methods such as magnetic resonance imaging (MRI) is extremely challenging and does not accurately reflect tumor burden. Furthermore, MRI is not a routine procedure due to the high costs involved. The alternative detection methods used in systemic cancers such as tissue biopsies are not possible in brain cancer without putting the patient’s life at risk.
This proposal aims at addressing these challenges by developing a minimally invasive liquid biopsy procedure that can be used for longitudinal monitoring of glioblastoma progression. The diagnostic power of circulating DNA released by brain tumor cells in the blood of glioblastoma patients will be evaluated using specific modifications of circulating DNA to precisely detect tumor burden. The development of such innovative, cost-effective, and safe techniques will transform glioblastoma patient management and provides a convenient way of monitoring tumor progression and treatment response. We eventually hope to develop an assay integrated with standard imaging techniques for minimally invasive monitoring of glioblastoma.
Discovery Grant
A Discovery Grant is a one-year, $50,000 grant to support cutting-edge, innovative approaches that have the potential to change current diagnostic or treatment standards of care for either adult or pediatric brain tumors.
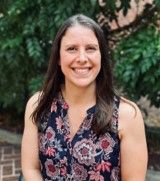
Theresa Barberi, PhD
Institution: Johns Hopkins University School of Medicine
Mentor: Alan Friedman, MD
Tribute: In memory of Kelli McLaughlin
Glioblastoma is a highly aggressive brain cancer, with less than 5% survival at five years. NF-kB p50, or simply “p50”, is a protein whose absence renders myeloid cells, white blood cells that are part of the immune system, more inflammatory due to its inhibitory effects on genes that control inflammation. We found that immature myeloid cells (IMCs) lacking p50 are active against glioblastoma in mouse models. Once infused, these “p50-IMCs” travel to tumors, develop into mature myeloid cells, and activate other immune system cells (including T cells) to fight the cancer.
STAT6 induces anti-inflammatory genes. We expect that myeloid cells lacking both p50 and STAT6 will be even more inflammatory than cells only lacking p50 and thereby will possess an even greater ability to cure glioblastoma. We will compare the ability of IMCs lacking p50 only, STAT6 only, and cells lacking both p50 and STAT6 (called a p50/STAT6 double knockout) to eliminate glioblastoma tumors in mice. We will also monitor mice for any toxicities; we have seen none thus far with p50-IMCs. In addition, we will develop p50/STAT6 double knockout in human cells and characterize them to learn if they are suitable for use in patients.
Upon completion of these studies, we anticipate rapidly evaluating our novel p50/STAT6 double knockout immature myeloid cell immunotherapy in clinical trials with the goal of increasing rates of cure and reducing toxicities of therapy for patients with glioblastoma and other high-grade brain cancers.

Defne Bayik, PhD
Institution: Sylvester Comprehensive Cancer Center, University of Miami Health Systems
Mentor: Antonio Iavarone, MD
Tribute: Fully supported by an Anonymous Family Foundation
The crosstalk between the tumor cells and the surrounding noncancerous cells drives the aggressiveness of glioblastoma (GBM), which is the most frequent and deadly malignant brain tumor. The treatment strategies aiming to kill tumor cells have not markedly improved patient outcomes over the past few decades. Therefore, understanding the complex interactions between tumors and their environment is important for the development of more effective anti-cancer therapies. One promising strategy is to educate the immune system to fight against tumors. However, tumors employ multiple mechanisms to recruit inhibitory immune cells, such as myeloid-derived suppressor cells (MDSCs), that would support the growth of the tumors.
We previously reported that different types of MDSCs play a role in the progression of GBM in males versus females. Recently, we observed that γ-aminobutyric acid (GABA), a critical modulator of neurons, can also act as a potential regulator of female-specific MDSC activity. In this project, we aim to understand how GABA reprograms MDSCs in females, and whether we can therapeutically target the pathways activated by GABA to reverse MDSC-mediated tumor growth. This project will identify new therapeutic opportunities for GBM that account for differences between males and females. Given that several GABA pathway inhibitors are commonly used in patients with mood disorders, repurposing these drugs can have an immediate impact on GBM outcomes.

Phedias Diamandis, MD, PhD
Institution: University Health Network
Tribute: Fully supported by An Anonymous Family Foundation
Cancerous brain tumors are challenging to detect and treat partly owing to the lack of early objective patient symptoms. In other cancer types (e.g. breast/colon) early detection through active monitoring has proven effective in improving patient outcomes. These approaches are, however, difficult to implement for less common cancers like glioblastoma (the most common/aggressive brain cancer). Recently, the application of advanced analytical methods like electroencephalography (EEG), a technique that allows monitoring of the brain’s electrical activity using external scalp electrodes, has re-emerged as a powerful clinical tool to non-invasively monitor/diagnose brain disease. Similar to the use of smartwatch devices to detect abnormal heart rhythms in the general population, it may now also be possible to use the growing number of consumer-grade EEG devices, commonly used for meditation and focus, to remotely detect brain tumors earlier in their evolution. Early detection could afford more effective treatments/outcomes. Our group has begun developing software to capture and analyze EEG data directly from patients/participants’ homes. This study is to determine how feasible/reliable remote EEG data collection is and if we can detect activity changes in patients with brain tumors, as their disease evolves/progresses. If successful, this EEG-based strategy bears potential value as a remote screening tool for early detection of brain tumors, in both the at-risk/general population.

Siddharthra Mitra, PhD
Institution: University of Colorado Denver
Tribute: In memory of Katie Monson
Brain tumors are among the deadliest cancers in humans. In adult Glioblastoma Multiforme (GBM) the average survival time is 12-18 months – only 25% of glioblastoma patients survive more than one year, and only 5% of patients survive more than five years. Similarly, pediatric high-grade gliomas are among the most deadly childhood cancer, with <20% of kids living past five years of diagnosis. One way to target brain tumor cells is by using the body’s own immune system against the tumor. One of the main immune cells that you find in your body is cells called macrophages, which essentially are like scavengers that go around eating dead or dying cells, infective bacteria and cells which have been infected with viruses. Cancer cells are known to protect themselves using proteins that tell immune cells not to attack them. Previously, we have published a “don’t eat me” signal on the surface of all the tumor cells, but if you block that “don’t eat me” signal, these macrophages can eat the tumor cells.
In this proposal, we will engineer these macrophages to carry homing devices on their surface that can direct their attack specifically on tumor cells, guided by the signals on the surface of the tumor cells. CAR T cell therapy, a form of immunotherapy that uses specially altered T cells – a part of the immune system that fights diseases, including cancer involves the collection of a patient’s T cells and genetically reprogramming them in the lab to recognize markers on specific cell types in the body. Engineering a similar protein-based homing device on the surface of macrophages will give them the ability to hunt out tumors and eat them up.

Allegra Petti, PhD
Institution: Massachusetts General Hospital
Tribute: Fully supported by an Anonymous Family Foundation
Glioblastoma (GBM), the most common primary malignant brain tumor in adults, is a devastating disease. Treatment for GBM has evolved little in recent decades, probably because this tumor type is notoriously complex: Each GBM tumor is heterogeneous with respect to genetics, tumor cell state, and immune microenvironment. In particular, a new technique called single-cell RNA-sequencing (scRNA-seq) has revealed that each GBM is a mixture of different types of tumor cells, each with different biological properties. Consequently, each tumor is essentially multiple diseases, and it will probably be necessary to treat each patient with drug combinations targeted to that individual’s tumor. Although scRNA-seq has enabled us to understand the complexity of GBM, it has not yet helped us treat the disease. This is partly because it is difficult to physically isolate and study the cell types discovered using scRNA-seq. Here, we propose a novel approach to isolating these GBM tumor cell populations, studying their therapeutic vulnerabilities, and identifying personalized drug combinations that target multiple cell populations simultaneously. Our approach is based on a new technology called CITE-seq, an extension of scRNA-seq that will enable us to define tumor cell populations and, in subsequent work, isolate them for further study using a technique called flow sorting.

Soma Sengupta, MD, PhD
Institution: University of North Carolina at Chapel Hill
Tribute: Fully supported by an Anonymous Family Foundation
In advanced cases, lung cancer that goes to the brain is a fatal disease. Treatment often uses radiosurgery, but this does not produce a durable response. We have a new drug (AM-101) that gets into the brain efficiently and can help the tumor microenvironment (the non-tumor cells and structural components in and around the tumor) become more favorable to treatments by increasing the population of an immune cell called a macrophage. What is unique is that the macrophage promoted by AM-101 kills cancer cells. We also know that AM-101 makes lung cancer cells more sensitive to radiation without side effects such as bone marrow toxicity. Once we complete these experiments, we intend to publish the data, and apply for R01 funding.

Daniel Silver, PhD
Institution: Cleveland Clinic
Mentor: Justin Lathia, PhD
Tribute: In memory of Chip Greenlee
Glioblastoma (GBM) remains among the most lethal of human cancers despite ongoing efforts to understand the mechanisms used by the tumor cells that define this cancer. The challenge is that GBM is far more than a collection of cancer cells independent from the brain. GBM invades extensively throughout the organ where it intermingles with numerous brain cells. The field has detailed several processes by which nerves and immune cells support the growth of this tumor. However, even though GBM tumors infiltrate fields of astrocytes, the most abundant cell type in the brain, we know far less about GBM-astrocyte interactions. Most mouse models of GBM develop within a capsule formed by highly reactive astrocytes. However, astrocytes do not demonstrate this wall-building behavior in human GBM. We do not understand the molecular signals that shield invasive GBM cells from astrocyte encapsulation in the human brain, nor do we fully understand the mechanisms and effects that result from GBM-astrocyte interaction. In this project, we aim to clarify the functional consequences of astrocyte-GBM interactions. Doing so will help close this major knowledge gap in the field and, more importantly, this project will produce a roster of factors that we will then evaluate as potential therapeutic targets against this devastating cancer. More generally, emphasizing astrocyte-tumor interactivity and revealing the signals that govern this relationship will help shift our perspective towards a more complete understanding of GBM as a disease of the brain, not simply a disease that resides in the brain.

Elizabeth Sweeney, PhD
Institution: The George Washington University
Mentor: Conrad Russell Young Cruz, MD, PhD
Tribute: In memory of Stephanie Lee Kramer
Glioblastoma (GBM) is the most commonly diagnosed brain cancer in the United States, impacting ~12,000 people per year. Despite intense standards-of-care that include surgery, radiation, and chemotherapy, the prognosis for GBM remains dismal, with a relative five-year survival rate of 7.5%. In response to the urgent need for effective therapies for patients with GBM, we are proposing a fundamentally new and personalized therapeutic approach that uses nanotechnology to train a patient’s own immune cells to recognize and kill their tumor cells. Our strategy involves applying heat to GBM cells that have been removed during surgery, which kills them in a way that can optimally engage with immune cells. Then, the heat-killed GBM cells are added to a dish containing immune cells from the same patient, which educates the immune cells to recognize the tumor cells. These immune cells are grown in the laboratory and then infused back into the patient as therapy. This platform is personalized to a patient’s own unique tumor makeup and as such, it allows the immune cells to recognize multiple potential targets (known and undiscovered) particular to a given patient. If successful, this project will lay the foundation for a new process for developing better adoptive immune cell therapies for treating brain cancer. As the nanoparticle-based platform can be applied to any cancer type, we envision its utility in developing effective therapies for additional brain and solid tumors.
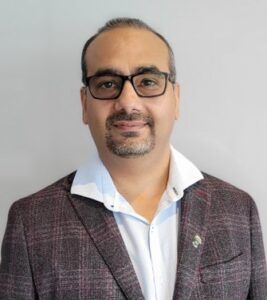
Nehalkumar Thakor, PhD
Institution: The University of Lethbridge
Tribute: Partially supported by Brain Tumor Foundation of Canada
Glioblastoma (GBM) is one of the deadliest cancers with a dismal prognosis and survival rate. Frontline therapeutic agents are failing in the clinic and there is an urgent need to develop novel therapeutic intervention strategies for the treatment of patients with GBM tumors. Under the stress conditions found within tumors, the normal (canonical) process in cells of translating genetic messages (mRNA) into protein is reduced to conserve cellular energy. At the same time, the cells begin the non-canonical process of translating a subset of mRNAs to different proteins so that the cell can cope with the stress condition. This non-canonical process contributes to the resistance of cancer cells against therapeutic agents. Proteins in cells known as eIFs have been shown to regulate the early steps of non-canonical translation of mRNA to protein. We have identified a protein, eIF5B, that facilitates the translation of anti-death proteins and allows GBM cells to survive during high-stress conditions that would normally cause cell death. We propose to define the role of eIF5B in GBM cell survival, proliferation, and non-canonical translation using brain tumor-initiating cells from patients. These experiments will validate eIF5B as a therapeutic target for GBM. This proof-of-concept project will establish the preclinical rationale for targeting eIF5B for the therapeutic benefit of GBM patients.
2022 Ongoing and Recently Completed Projects
Discovery Grant
A Discovery Grant is a one-year, $50,000 grant to support cutting-edge, innovative approaches that have the potential to change current diagnostic or treatment standards of care for either adult or pediatric brain tumors.

Osama Al-Dalahmah, MD
Institution: Columbia University
Mentor: Peter D. Canoll, MD, PhD
Tribute: Fully supported by the Uncle Kory Foundation
Glioblastoma (GBM) is a devastating malignant brain tumor that defies surgical resection and traditional therapies. Despite the advances in GBM management, patient survival is dismal because there are no effective therapies. Recent efforts examined targeting cells other than GBM cells, mainly immune cells, as a potential treatment for GBM, but this approach was ineffective. This may be due to the nature of the cells that reside in the periphery of the tumor resected by the neurosurgeon (resection margin), which consists of few tumor cells and immune cells, and many neurons and astrocytes.
Astrocytes are the major support cells in the brain that maintain the health and function of other brain cells. Evidence suggests that astrocytes contribute to GBM spread and growth. My preliminary data suggests molecules that come from astrocytes can promote GBM progression. In this study, I hypothesize that eliminating these molecules, and thereby blocking the interaction between the astrocytes and GBM cells, will lead to reduced GBM spread, increased GBM cell death, and may make GBM more vulnerable to therapy. I will test this hypothesis in animal models of GBM, patient derived GBM tissue, and cellular models of GBM using a combination of cutting-edge molecular studies designed to test GBM cell function and gene expression. My project has the potential to uncover novel therapies for GBM based on targeting astrocytes and may provide neurooncologists with additional tools to combat GBM.
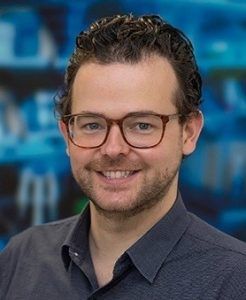
Floris Barthel, MD
Institution: The Translational Genomics Research Institute
Mentor: Michael Berens, PhD
Tribute: In honor of Joel A. Gingras, Jr.
By catching glioma progression early, we can treat it faster and keep it under control. Glioma follow-up generally consists of repeated MRI-scans and physical examination. Unfortunately, by the time a new tumor is detected, the relapsed tumor already consists of billions of cells, making treatment needlessly difficult. There is thus a critical need for new techniques that can detect recurrence earlier than currently possible.
There is now immense opportunity in developing technologies capable of detecting recurrence from liquid biopsies such as from a drop of blood. The majority of DNA is stored in the nucleus of a cell and one promising strategy to predict recurrence is to search for fragments of tumor DNA circulating in the blood using DNA sequencing. Unfortunately, studies using DNA from the nucleus to predict recurrence in glioma have not been successful. However, a separate piece of DNA is stored in mitochondria, which are cellular organelles (organs of the cell) that function to provide energy. In contrast to nuclear DNA, mitochondrial DNA has a number of properties making much more likely to be detected circulating in blood. Using circulating mitochondrial DNA as a marker to track cancer burden is therefore incredibly promising. If this research is successful, it will revolutionize the care of glioma patients by translating into new technologies that can detect tumor recurrence early, effectively increasing patient lifespan and quality of life.
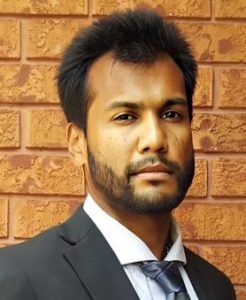
Andrew Beharry, PhD
Institution: University of Toronto
Mentor: Sunit Das, MD, PhD
Tribute: Partially supported by Brain Tumor Foundation of Canada
Gliomas are a classification of primary brain tumour. The standard of care often begins with surgical resection whereby the surgeon uses pre-operative scans (e.g., MRI) as a guide to identify the cancerous tissue that should be removed. However, as tissues are constantly changing, using a single diagnostic image for surgery can be challenging and so surgeons often depend on their sight and touch, which leads to uncertainty in tumour margins, causing cancerous tissue to remain post-surgery, or the removal of healthy tissue, which could be detrimental to the patient. A solution to overcome these issues is to use fluorescence guided surgery (FGS) which uses small molecules called fluorophores that target cancer cells to enhance the real-time detection of tumours during surgery. In the context of existing research and treatment, a molecule called 5-aminolevulinic acid (5-ALA) has been used for FGS but is only effective for high grade gliomas and not for guiding the resection of lower-grade, deep-seated tumors. Our project describes the development of the first fluorescent chemosensor for a mutant form of isocitrate dehydrogenase 1 found in 80% of adult low-grade gliomas. Our proposed sensor will produce red fluorescence in gliomas and yellow fluorescence in healthy tissue, thereby permitting rapid identification of gliomas over healthy tissue of patients in the operating room. If successful, clinicians around the world can routinely employ the sensor for FGS, reducing recurrences post-surgery, thereby improving patient survival rates.

Aashim Bhatia, MD
Institution: The Children’s Hospital of Philadelphia
Mentors: Timothy Roberts, PhD, and Michael Fisher, MD
Tribute: Fully supported by An Anonymous Family Foundation
Currently the standard approach of monitoring brain tumors includes radiology imaging, such as MRI. These clinical MRIs provide important information for the clinical teams but have many limitations in knowing if a cancer treatment is working. We are researching an MRI approach to looking at sodium levels in the brain and brain tumors, since we know brain tumors have increased sodium levels based on our preliminary data and the literature. However, much more data is needed and there are no research studies in children. Diffuse midline gliomas are highly aggressive brain tumors that are treated with radiation. After radiation, the goal is the tumor shrinks in size, but radiation changes can make the tumor look like it is enlarging, when actually the tumor itself is getting smaller, and we currently don’t have an easy way to determine this with clinical MRIs. Biopsy cannot be performed at multiple timepoints during treatment due to the risk of complications. We believe measuring the sodium values in tumors with this advanced imaging technique (Sodium MRI) proposed in this grant will help understand the behavior in brain tumors that cannot be obtained with the current clinical MRIs. If successful, this advanced MRI technique will make an accurate diagnosis of tumors that are responding or not responding to treatments, allowing doctors to make treatment decisions quickly to improve outcomes in children with brain tumors.

Henk De Feyter, PhD
Institution: Yale University
Tribute: In memory of George Surgent
Magnetic resonance imaging (MRI) plays a critical role in guiding treatment of patients with glioblastoma, the most aggressive and lethal brain tumor. MRI scans can provide exquisite detail about the location and shape of a brain tumor. However, from MRI scans we cannot immediately tell if a tumor is growing or not. Instead, multiple MRI scans are needed over several months, to see if a tumor is changing in size. Even this can be challenging because cancer treatments can often lead to changes on the MRI scans that appear as if a tumor is growing when in reality, it is not. This uncertainty about tumor growth makes it complicated for doctors to design the best treatment plan and can lower patients’ quality of life.
We recently developed a new MRI-based technology (deuterium metabolic imaging, DMI) that can image the fuel consumption of a brain tumor. Before DMI scans, patients are given a safe, non-radioactive form of sugar, dissolved in water that they drink. One hour later the DMI scan is performed to visualize the high sugar consumption that is typical for these aggressive tumors. This new DMI technique could make it possible to detect to what degree a tumor is still active, in a single scan.
In this project we propose to test if DMI is indeed good at detecting growing tumors in patients with glioblastoma, by comparing DMI with resected tumor tissue. Both DMI scans and tumor tissue are collected from patients that are participating in a therapeutic clinical trial. If successful, DMI will allow doctors to make informed decisions about treatments earlier in the progression of disease and will hopefully lead to better outcomes for patients.
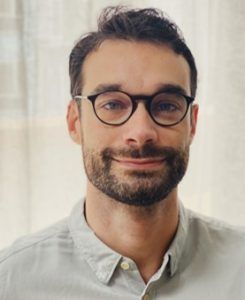
Federico Gaiti, PhD
Institution: University Health Network
Mentor: Gelareh Zadeh, MD, PhD, FRCSC, FAANS
Tribute: In honor of Joel A. Gingras, Jr.
Tumors continuously evolve towards more aggressive and resistant forms. Brain tumors illustrate the problem of cancer evolution. Despite standard-of-care treatment, many of these tumors inevitably recur. Glioblastoma (GBM) is the most aggressive and common type of primary malignant brain tumor, with a survival rate averaging about one year. Thus, there is an urgent need for novel treatments.
Our preliminary work suggests that these incurable brain tumors display a large diversity among tumor cells both within and between tumors. Identifying the key factors that underlie this cellular diversity that leads to the conversion of normal cells to cancer cells is therefore a critical goal with broad implications for oncology. Towards this goal, we seek to apply innovative genomics and experimental technologies to unique brain tumor samples. These findings will allow us to better understand differences between individual malignant cells and identify the molecular factors that promote brain tumor cells growth and therapeutic resistance.
We envision that these factors can be exploited to devise novel treatment strategies designed to limit the evolutionary potential and growth of these lethal tumors, ultimately improving patient outcomes. We further anticipate that the approaches generated in this work will be broadly applicable to other brain tumor types, yielding novel frameworks to address the central challenge of brain tumor evolution.
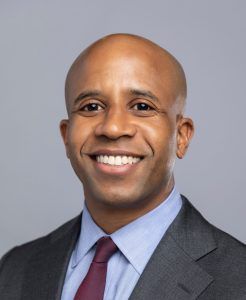
Edjah Nduom, MD
Institution: Emory University
Tribute: Fully supported by Southeastern Brain Tumor Foundation
Patients suffering from glioblastoma desperately need new treatment options. We have identified a combination immunotherapy that could provide a critical breakthrough. Checkpoint inhibitors are a new type of cancer immunotherapy that has revolutionized cancer treatment. Cancer cells are smart and prevent the immune system from attacking them by stimulating “checkpoints” that tell the immune system to stand down. Checkpoint inhibitors block these immunosuppressive checkpoints and restore the ability of the immune system to attack cancer directly. Unfortunately, checkpoint inhibitor therapy has not worked well enough for patients suffering from glioblastoma. Scientists believe this is due to a population of cells called macrophages that keep active immune cells out of the tumor. A promising new treatment, OS2966, could block these macrophages and enable checkpoint inhibitors to work in glioblastoma. OS2966 has been tested in mice, and it shows activity against glioblastoma. Also, OS2966 is already being evaluated for safety in patients in a phase 1 trial for patients with recurrent glioblastoma (NCT04608812).
In this project, we will test OS2966 together with pembrolizumab, a checkpoint inhibitor that is FDA approved for other cancers. We will determine if these drugs can activate immune cells grown in dishes with glioblastoma cells. We will also treat mice with brain tumors using these immune therapy drugs. After successfully completing these studies, we will be able to rapidly bring these drugs to clinical trials for patients.

An-Chi Tien, PhD
Institution: Barrow Neurological Institute – St. Joseph Medical Center
Mentor: Nader Sanai, MD, and Shwetal Mehta, PhD
Tribute: Fully supported by An Anonymous Family Foundation
Meningiomas are the most common primary brain tumors with approximately 35,000 new cases each year in the United States. Among them, 20% of meningiomas are aggressive and there is no FDA-approved drug treatment for this population, thereby patients suffer from repeated surgical resections and radiotherapy and the long-term side effects that result from these treatments. The Ivy Brain Tumor Center has initiated a phase 0 clinical trial to investigate a promising drug, ribociclib, that aims at dampening tumor growth, one of the hallmarks of meningioma cells. While the clinical trial is showing early signs of promising clinical effect, we have identified patients that respond to the treatment for a long period of time, representing the ‘responder’ patients who stay progression-free for a median of 22 months.
In this proposal, our goal is to identify a common genetic signature in the responder patients that will help identify patients who are likely to benefit from this treatment. The signature that we identify from this study will be validated in a larger phase 2 study to develop companion diagnostic biomarkers for this drug. Furthermore, we propose to use a patient-derived meningioma mouse model to understand how meningioma cells become resistant to ribociclib in ‘non-responder’ patients and seek to strategize future combination treatment options for them.
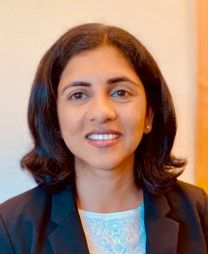
Pavithra Viswanath, PhD
Institution: University of California, San Francisco
Mentor: Susan Chang, MD
Tribute: Fully supported by An Anonymous Family Foundation
Gliomas are a pernicious form of cancer with long-lasting physical and cognitive deficits. Mutations in the metabolic enzyme isocitrate dehydrogenase (IDHmut) are the defining molecular feature of lower-grade gliomas. Unlike many cancers, obtaining biopsies repeatedly from glioma patients is difficult because of the complications of brain surgery. As a result, management of IDHmut glioma patients is dependent on non-invasive magnetic resonance imaging (MRI) techniques. However, because MRI does not highlight biological events critical for tumor growth, it does not reliably report on disease progression and response to therapy.
Deuterium Magnetic Resonance Spectroscopy (DMRS) is a non-invasive, non-radioactive method of imaging tumor metabolic activity. Our preliminary studies provide strong evidence for the ability of a non-radioactive molecule called deuterated alpha-ketoglutarate to monitor IDHmut activity in gliomas. In this proposal, we will validate the ability of deuterated alpha-ketoglutarate to determine tumor burden and early response to therapy in preclinical IDHmut glioma models. Clinical translation of our studies is possible within 2-4 years and will provide clinicians with a novel precision imaging tool for monitoring IDHmut glioma patients.

Jacky Yeung, MD
Institution: Yale University
Mentor: Lieping Chen, MD, PhD
Tribute: Fully supported by An Anonymous Family Foundation
Meningiomas are the most common primary intracranial tumors with a prevalence of ~170,000 cases in the United States. While surgical resection is often curative for benign meningiomas, higher-grade tumors tend to recur. There is a clearly unmet need for alternative therapies. Tumor blood vessels may be a chemical and physical barrier for effector T cells (a type of immune cell) to reach and attack the tumors. Recently, our group identified a novel interaction between CD93, and its binding partner IGFBP7 two molecules that are found in high levels in tumor blood vessels but not normal blood vessels. We can target the interaction between these two molecules to improve drug delivery and increase immune cell infiltration into the tumors.
Our general hypothesis is that inhibition of CD93/IGFBP7 to achieve blood vessel normalization will increase T cell infiltration for the treatment of malignant meningiomas. This proposal addresses this unmet need in the treatment of malignant meningiomas as there are limited options currently for patients who have exhausted surgical and radiation options.
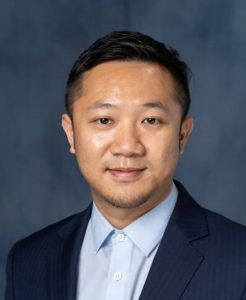
Fan Zhang, PhD
Institution: University of Florida
Mentor: Jeffrey Harrison, PhD
Tribute: Fully supported by StacheStrong
A major obstacle for successful immunotherapy for brain tumors is the hostile immune-suppressed tumor microenvironment (TME) that limits treatment efficacy. Tumor-associated macrophages (TAMs) are a diverse population of immune cells derived from various origins such as brain and bone marrow. These cells are the major immune cells that are found in gliomas. The TAMs promote tumor development and influence the effect of the standard-of-care cancer treatments and immunotherapies. Recent studies have indicated that different types of TAMs have different roles in promoting glioma development and influence treatment effect; therefore, these TAM subsets should be targeted separately. Unfortunately, current therapeutics to target TAMs do not differentiate between TAM subsets, and they also have toxicities and limited access to the tumor.
To address this limitation, we propose to develop a novel therapy to selectively target TAM subsets in brain tumors through intravenous infusion. Our previous study has established that this therapy can reach the brain tumor and selectively target TAMs in the tumor. Now, we need to investigate what subset(s) of TAMs are being targeted and whether this therapy would allow reduced side effects. Implemented into the clinic, this therapy will safely modulate TAMs and potentiate standard-of-care treatment or cancer immunotherapies for brain tumor patients. This interdisciplinary study will advance our understanding of how therapeutics interact with specific TAM subsets; and how modulate the TAM subsets can reshape the brain tumor microenvironment for more effective immunotherapy.
Basic Research Fellowship
The Basic Research Fellowship is a two-year, $100,000 grant, awarded to post-doctoral fellows who are mentored by established and nationally-recognized experts in the neuro-oncology field.

Kristin Huntoon, PhD, DO
Institution: University of Texas M.D. Anderson Cancer Center
Mentor: Betty Kim, MD, PhD
Tribute: Fully supported by StacheStrong
Cancer cells in glioblastoma can hide from the body’s immune system, thus allowing for the cells to continue growing. These cells can hide by expressing a surface molecule that makes the cells invisible to the immune system. Other therapies designed to activate the immune system against glioblastoma have been tested in clinical trials but have failed to demonstrate significant improvement in overall survival. This failure may be due to this ability of cancer cells to evade detection due to this molecule on the cell’s surface. In the proposed project, we will preclinically test our novel therapy, which is designed to unmask the invisible cloak on glioblastoma cells, allowing immune cells to detect and destroy them. The resulting tumor cell fragments stimulate immune signaling pathways, which further amplify immune responses against glioblastoma. We will evaluate the antitumor effect of this therapy against patient glioblastoma models established in mice which have a functional human immune system, thus potentially providing us with direct evidence of the therapeutic efficacy of this new treatment. As glioblastoma is difficult to treat, the proposed project can significantly propel the field of brain cancer research forward and directly aligns with future strategies of developing more effective therapies for glioblastoma.
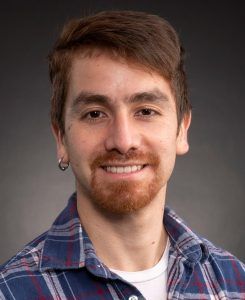
Jorge Ibanez, PhD
Institution: St. Jude Children’s Research Hospital
Mentors: Giedre Krenciute, PhD and Susanne J. Baker, PhD
Tribute: Fully supported by Humor to Fight the Tumor
The goal of this project is to develop antigen-specific T cells as an effective immunotherapy for diffuse intrinsic pontine glioma (DIPG), a deadly pediatric brain tumor resistant to radiation and chemotherapy. The immune defense system against cancer often fails because cancer can evade or actively inhibits immune response. However, genetic modification of the patient’s own immune system can be used to endow T cells with the ability to recognize and kill cancer cells. Genetically engineered T cells has shown dramatic success in clinical studies against leukemia, as well as promising results against solid tumors. We have now developed this treatment approach for pediatric patients with DIPG. In our methods, we have modified T cells to recognize and kill tumor cells that specifically have a molecule called B7-H3. These modified T-cells have demonstrated anti-DIPG activity in pre-clinical models. In this project we now propose to improve B7-H3-specific T cells anti-DIPG activity, by enhancing the interaction between T cells and tumor cells. This interaction is crucial as it mediates effective and sustained T cell antitumor response. First, we will characterize molecules that control T cell and DIPG cell interaction. Then we will knock-out RASA2, a molecule that weakens T cell and DIPG interaction and test if this modification improves T cell anti-DIPG response. If our pre-clinical approach is successful and a clinical study is justified, we have the resources to develop a Phase I clinical trial at our institution.
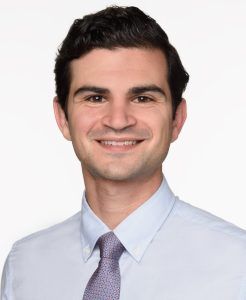
Aram Modrek, MD, PhD
Institution: New York University School of Medicine
Mentors: EriK Sulman, MD, PhD and Jane Skok, PhD
Tribute: Fully supported by Tap Cancer Out
Radiation therapy, like some chemotherapies, is thought to be effective at killing cancer cells because it damages the genetic material (DNA) of cancer cells within a tumor. However, the aftermath of radiation is extremely difficult to study because the damage to the DNA is random. An analogy of this would be to consider raindrops falling on a lake; we cannot predict where the next raindrop will fall, and we cannot predict how the hundreds of ripples they create may look from one moment to another. Similarly, radiation particles hit the cell’s DNA at random locations, causing a ripple effect of damage and a cascade of events in which the cancer cell repairs the damage. To circumvent the random nature of DNA damage by radiation so that we can better understand the consequences, we develop here a system that allows us to create hundreds of damaged DNA sites at the same time, and at pre-mapped locations. The preliminary data thus far has shown that using this approach allows us to study the “aftermath” of DNA damage with much greater clarity in glioblastoma than has previously been possible. For example, we can see how DNA mutations arise, and how the cell may leave chemical modifications or “scars” on the DNA that change how the DNA is interpreted by the cell. Ultimately, it is the goal of this fellowship proposal to fully develop these tools and learn more about the fundamental biology so that we may therapeutically target the aftermath of DNA damage to prevent glioblastoma from being able to recover from therapy and grow back.
Glioblastoma (GBM) is a highly malignant tumor derived from glial cells. It is the most common primary malignant in adults with a median survival of 15 months. Current treatment strategies involve surgical resection followed by radiotherapy with chemotherapy. Unfortunately, GBM is highly resistant to therapy and will inevitably recur resulting in poor patient outcomes. Deeper characterization of the tumor environment has revealed the presence of great diversity among the cells that make up the tumor, hypoxic regions (low Oxygen level), high cell growth or proliferation, and angiogenesis. The critical cellular player involved with these tumor behaviors is the glioblastoma stem-like cell (GSC).
Strategies to target these GSCs have proven to be unsuccessful so far but remain a promising avenue for research. There is a need to develop a deeper understanding of GSC biology, starting with the regulatory mechanisms with which they adapt to the hypoxic environment of GBM and promote tumor growth. However, most studies exploring GSCs are performed in normoxic (normal levels of oxygen) conditions. Further, the central aspect of translation, an important step in gene regulation where genetic information is converted to functional proteins, remains understudied. Our proposal involves studying the translation (mRNA-to-protein) regulatory step in protein synthesis, as opposed to the more commonly explored transcription (DNA-to-mRNA) regulatory step. We expect to find genes that are modulated by hypoxia exclusively through translational regulation, revealing a novel list of candidates for future therapy.
Meningioma is the most common tumor within the skull, arising from the tissue surrounding the brain. While many meningiomas are non-cancerous, up to 20% are pre-cancerous or cancerous (i.e., high-grade). Patients with high-grade meningioma have very bad outcomes despite treatment with surgery and radiation. There are currently no available medical treatments for high-grade meningioma, such as chemotherapy or immunotherapy. Immunotherapy, for example anti-PD1 immunotherapy, utilizes the immune system to target diseases, including tumors. However, research has found that tumors change their local surroundings to weaken the immune system, leading to tumor growth. Interleukin-1-beta (IL1ß) is a protein produced by tumors that weakens the immune system. Blocking IL1ß can restore the immune system, making immunotherapy more effective. Research in other cancers has shown that blocking IL1ß works well for females, but not males. This study will be the first to investigate whether females and males with high-grade meningioma have different responses to IL1ß blockade combined with anti-PD1 immunotherapy. The results of this study could lead to future clinical trials and ultimately the first approved medical treatment for high-grade meningioma.
Glioblastoma (GBM) is a highly malignant tumor derived from glial cells. It is the most common primary malignant in adults with a median survival of 15 months. Current treatment strategies involve surgical resection followed by radiotherapy with chemotherapy. Unfortunately, GBM is highly resistant to therapy and will inevitably recur resulting in poor patient outcomes. Deeper characterization of the tumor environment has revealed the presence of great diversity among the cells that make up the tumor, hypoxic regions (low Oxygen level), high cell growth or proliferation, and angiogenesis. The critical cellular player involved with these tumor behaviors is the glioblastoma stem-like cell (GSC).
Strategies to target these GSCs have proven to be unsuccessful so far but remain a promising avenue for research. There is a need to develop a deeper understanding of GSC biology, starting with the regulatory mechanisms with which they adapt to the hypoxic environment of GBM and promote tumor growth. However, most studies exploring GSCs are performed in normoxic (normal levels of oxygen) conditions. Further, the central aspect of translation, an important step in gene regulation where genetic information is converted to functional proteins, remains understudied. Our proposal involves studying the translation (mRNA-to-protein) regulatory step in protein synthesis, as opposed to the more commonly explored transcription (DNA-to-mRNA) regulatory step. We expect to find genes that are modulated by hypoxia exclusively through translational regulation, revealing a novel list of candidates for future therapy.
Meningioma is the most common tumor within the skull, arising from the tissue surrounding the brain. While many meningiomas are non-cancerous, up to 20% are pre-cancerous or cancerous (i.e., high-grade). Patients with high-grade meningioma have very bad outcomes despite treatment with surgery and radiation. There are currently no available medical treatments for high-grade meningioma, such as chemotherapy or immunotherapy. Immunotherapy, for example anti-PD1 immunotherapy, utilizes the immune system to target diseases, including tumors. However, research has found that tumors change their local surroundings to weaken the immune system, leading to tumor growth. Interleukin-1-beta (IL1ß) is a protein produced by tumors that weakens the immune system. Blocking IL1ß can restore the immune system, making immunotherapy more effective. Research in other cancers has shown that blocking IL1ß works well for females, but not males. This study will be the first to investigate whether females and males with high-grade meningioma have different responses to IL1ß blockade combined with anti-PD1 immunotherapy. The results of this study could lead to future clinical trials and ultimately the first approved medical treatment for high-grade meningioma.